Cranes, wind-turbine blades, and airplane wing flaps all have a similar problem: The bearings that help them rotate don’t go in a full circle. Like kids on a swing or seesaw, they oscillate back and forth or up and down.
Some oscillating bearings also can be thought of as carrying a load rather than rotating a device, said Mike Chartre, CEO of Powertrain Engineers, a company that designs industrial gearing shafts and bearing systems.
“It’s a static bearing being used for position, not for rotation,” he said. “It’s transmitting a torque, typically when you have an application like wind-turbine blades. It’s like a Lazy Susan bearing on your spice rack that you turn to the position you need. The purpose of the bearing isn’t so you can spin it around in circles.”
Spinning Lazy Susans aside, with so many cycles happening in applications that don’t lend themselves to easy replacement, understanding this motion and the damage it can cause is critical to predicting an accurate lifetime for bearings in oscillating applications. Not only is this type of motion severe and demanding but so is the starting and stopping as the rotation reverses.
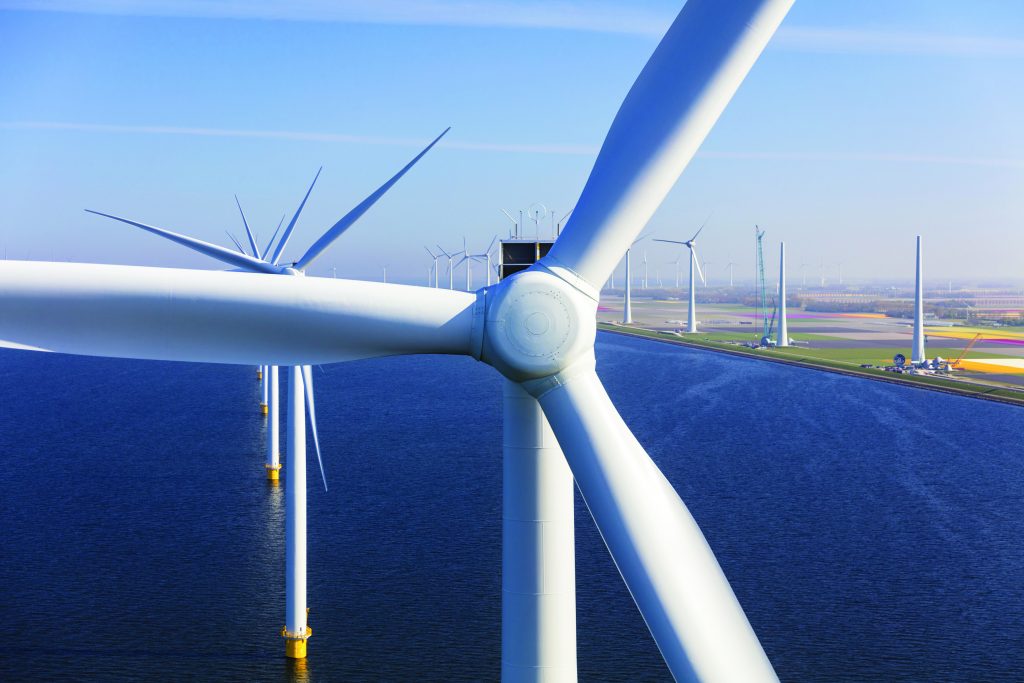
“The standards of the bearing world are all set up around the full rotation of a bearing; there are changes to those calculations that happen with oscillatory motion,” said Ian Hegner, manager of research and development at Rexnord Corp., a company that designs applications for the airplane industry such as wing and tail flaps.
Design engineers looking to determine the lifetime of rotational bearings use analyses from 1947 that made the basis for a 2007 standard known as ISO 281 [1]. Since then, engineers have developed modifications to that standard that consider oscillations rather than full rotations, but different assumptions and approaches overestimate lifetimes or fatigue, and the size of the oscillation also must be taken into account [2,3].
Also, conditions in real life aren’t usually ideal. In an analysis in 2018 of a crane winch [4], the authors pointed out that unlike that kid on the swing, real oscillations in the crane are imperfect and bumpy rather than a smooth sine curve. The approximations differ enough from reality that the equations might be overestimating the lifespan of oscillating bearings.
Angling Toward Lifespan
Lack of big movement damages bearings in propellers, turbines, and other applications slowly and indirectly. For example, in a 2018 computer simulation of a wind turbine, researchers showed that the pitch bearings — the ones that let the blades twist on their long axes to catch the right amount of wind — are at risk of surface-induced damage by oscillating between 0 and 2.5 degrees for about 127 successive days over 20 years [5].
“While they’re not spinning around in the traditional sense that you think of in a bearing, the bearing elements have little movement and potentially a ton of force,” Chartre said. “And if it just moves back and forth a little bit, eventually it’ll evacuate all the grease out of the raceway. And once the grease is gone, there’s no film to protect the surfaces from adhesion. So, on the microscopic level, you get really high loads and, potentially, welding of the two surfaces together for an instant. And then it breaks apart when there is movement. That can happen over and over, and eventually you get degradation of the surface. That’s called fretting.”
Engineers find that rotating bearings are a place to start to determine lifespan. The simplest way to determine the lifespan of bearings in oscillating applications is to sum up the distance the oscillations move, convert that total into full rotations, and use that in the standard ISO 281 [5].
“That’s something that everybody agrees on pretty universally until you get below that critical angle,” Hegner said.
Small angle oscillations create different damage conditions than full rotations. Rotational bearings are subject to rolling contact fatigue. They are rotating long distances (relatively) and at high speed under elastohydrodynamic conditions. But elastohydrodynamic conditions disappear with small amplitudes, slow speeds, and heavy loads. These conditions create surface-induced damage such as false brinelling and fretting.
Enter the critical angle, one of two relevant angles to consider to understand the damage modes. In seminal work as a lifetime member of ASME, the late John Rumbarger provided commonly used definitions: The critical amplitude of oscillation is defined as the angle of rotation of one race relative to the other race for which the race path stressed by one rolling element, such as a ball or roller, just touches but does not overlap the race path stressed by adjacent elements [6].
Another zone of operation called the dither zone is oscillation at very small amplitudes where the stressed area, or contact footprint between the element and the race, is only partially uncovered and then retraced [7].
“As you leave that critical angle and get toward the dither angle, it’s a whole different equation to determine the life of a bearing.” Hegner said. “And that’s the area that needs more attention, in my opinion, because the bearing world is more concerned about fatigue and whole rotation. These oscillatory application engineers are more worried about the wear piece of it, and that’s the more difficult piece to get your hands around.”
Barely Moving and the Damage Done
One way the simple back and forth movement going very small distances causes damage is by starving the bearings for lubrication. Small angles don’t push the grease around enough. And different amplitudes create different kinds of damage.
“If it’s a larger angle, which would be around the critical angle or larger, you may see metal-to-metal contact, and that would likely create surface-initiated spalling failure,” Hegner said. “And if it’s a small angle that’s closer to dither, that would be your fretting failure.”
“Other kinds of oscillations can cause damage as well,” said Ed Hahlbeck, CEO and owner of Powertrain Engineers. “In applications where the load is oscillating and the bearing is basically static, the wear occurs due to the changing contact position as load changes. Thus, very low internal clearance is critical, along with adequate support to keep races aligned.”
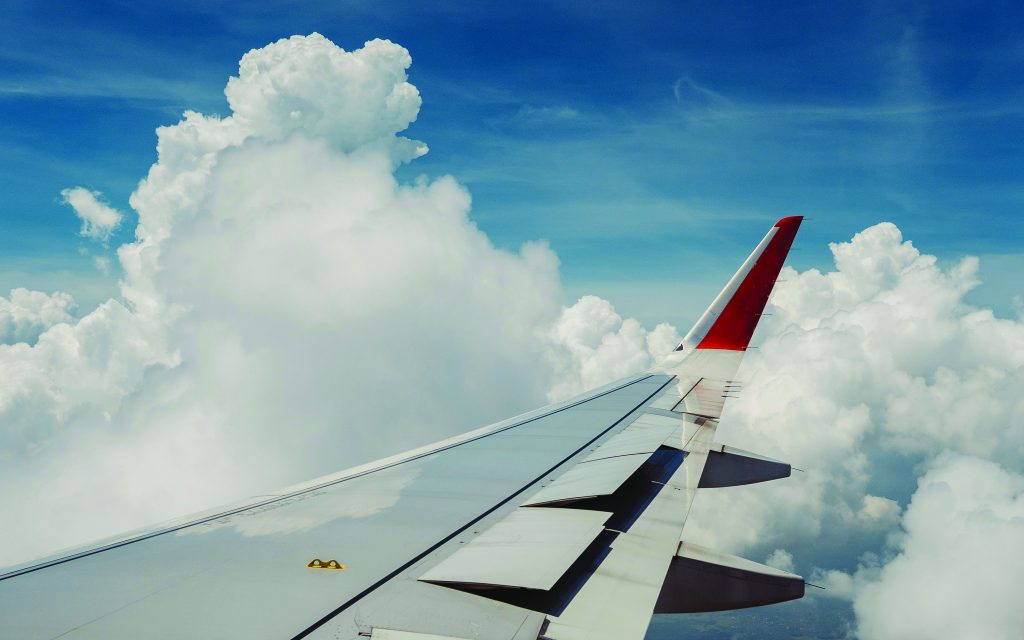
If the raceways and elements are starved for lubrication and grind past each other, they can create shallow ruts known as false brinelling. True brinelling deforms metal into pits or ruts, but false brinelling can involve some loss of metal. Under the right conditions, say if water gets into the bearings, these ruts can grow, deepen, and turn into fretting corrosion.
Another type of failure that doesn’t have to do with lack of lubrication, but with the hardness between, is rollers and raceways.
“If you have a really hard roller against a softer inner ring, and you just have the small back and forth movement, you can actually create a little groove in a deforming way,” Hegner said. “It’s kind of like fretting, but it’s wear due to a hardness differential.”
To counteract the potential damage, design engineers sometimes make the rolling elements cover more space.
“One way is to go for broader surfaces, using something other than just a ball,” Chartre said. “Cross roller designs are pretty common in these sorts of applications.”
The elements in cross roller bearings look like barrels. They’re tipped on their side 45 degrees, with the next one tipped the other way at 45 degrees. The roller touches the raceway along a length rather than on a point like balls do. For example, if you roll a ball along the ground, the ball touches the ground at one point. With a bearing, this forms a line in the middle of the raceway as the ball rolls. If you tip a barrel on its side and roll it on the ground, the whole length of the barrel touches the ground, from top to bottom. And so on the raceway, a roller bearing spans more of the raceway than a ball bearing, distributing wear more widely.
Some turbines have automated systems that can tell when the wind dies down enough to give blades a good twist, just to spin the bearing every so often.
Feeding Lubrication
Engineers discovered damage due to false brinelling, sometimes considered fretting, due to oscillations almost 100 years ago. Back in the 1930s, automobiles that shipped by train ended up with damaged wheel bearings. The vehicles were tied down on train cars, but they still jostled on the rickety tracks. Like Hahlbeck’s example of an oscillating load and a static bearing, the captive wheel bearings suffered from unwanted contact.
“To combat that, there are anti-fretting additives in greases, so you can modify the lubricant,” Hegner said. “In our industry and aerospace, our customers pick one or two different greases, and we’re at the mercy of what they pick.”
For today’s oscillating bearings, guidelines suggest, at a minimum, using grease with anti-wear and extreme-pressure additives [8].
Design methods are a possible way to disperse the lubrication throughout. Evenly spacing input points around the bearing and putting grease exit openings between them is one idea.
“In this way, the grease has a short distance to travel to cover the areas and can expel the residual grease nearby,” Hahlbeck said. “Often this type or application will generate some frettage debris, thus expelling used grease is critical.”
Rexnord designed a “precessing retainer” to make the elements move around the raceway, albeit slower than in rotational bearings. A slight tilt in the guide ring for the rollers moves the bearings “five steps forward and four steps back.” [9]
“It is sort of like a one-way clutch, but it doesn’t totally restrict movement,” Hegner said. “It moves easier in one direction than the other, and that will move the rollers all the way around the bearing, which will then in turn load all the rollers and then also load different areas in a ring. So, it just distributes that wear throughout the bearing.”
Hegner said the precessing retainers work well under small-angle applications.
“If we have an application that has a high amount of dither cycles, then we would recommend the precessing retainer when we design the part,” he said. “If it has a high amount of large angle oscillations, then we wouldn’t recommend it because it would create too much resistance in the motion. Under very small angles, plus or minus five degrees or less, it’s a successful type application. But if a significant amount of the application’s cycles are higher than five degrees, then the precessing retainer might become more of a detriment than a benefit.”
Another method is to just spin the bearing every so often.
“In the case of turbines, it is important to pitch the bearings or to execute a yaw movement to redistribute the lubricant,” Hahlbeck said. Some turbines have automated systems that can tell when the wind dies down enough to give blades a good twist.
“The primary direction of the wind is largely the same every day,” Chartre said. “But every once in a while, when the wind allows, they’ll spin the thing around 360 degrees one way and spin it back the other way.”

Future Spins
Advancements in greases, coatings, or surface treatments might be in the future to prevent damage to oscillating bearings or lengthen their life.
“I think we’re seeing more and more of the small angle applications in our industry,” Hegner said. “We’ve been very successful with the precessing retainer, but I think there are still more solutions out there.”
For applications in which the load is almost always in one spot, such as building cranes, crawlers, or mining shovels, remounting is a way to extend the life of a bearing.
Improved additives for greases would help some industries, although Hegner said the aerospace industry can be slow to adapt to new things because of safety issues.
Up in space, new coating or surface advancements would be better than ones in grease since greases don’t work due to a lack of oxygen. However, airplanes have very high loads that can crack away a coating.
Low-tech solutions also are out there. For applications in which the load is almost always in one spot, such as building cranes, crawlers, or mining shovels, Chartre suggests remounting as a way to extend the life of a bearing.
“One of the things that you can do on a manual application is if you have a bearing that is going to be oriented one way for most of its life, you could probably extend the use of that bearing by occasionally remounting it, so it’s now in a new position sharing a load somewhere else,” Chartre said.
“You could get really get clever and build a system to rotate the bearing, which is probably going to involve another bearing.”
REFERENCES
- ISO (2007), “ISO 281:2007 Rolling bearings —Dynamic load ratings and rating life,” International Organization for Standardization. Available at https://www.iso.org/standard/38102.html.
- Rumbarger, J. (2003), “Simplification of dynamic capacity and fatigue life estimations for oscillating rolling bearings,” ASME Journal of Tribology, 125 (4), pp. 868-870. Available at https://asmedigitalcollection.asme.org/tribology/article-abstract/125/4/868/462260/Simplification-of-Dynamic-Capacity-and-Fatigue.
- Wöll, L., Jacobs, G. and Kramer A. (2018), “Lifetime calculation of irregularly oscillating bearings in offshore winches,” Modeling, Identification and Control, 39 (2), pp. 61-72. Available at https://www.mic-journal.no/ABS/MIC-2018-2-2.asp/.
- Ibid.
- Stammler, M., Reuter, A. and Poll, G. (2018), “Cycle counting of roller bearing oscillations – case study of wind turbine individual pitching system,” Renewable Energy Focus, 25, pp. 40-47. Available at https://www.sciencedirect.com/science/article/pii/S1755008417302624.
- Rumbarger, J. (2003), “Simplification of dynamic capacity and fatigue life estimations for oscillating rolling bearings,” ASME Journal of Tribology, 125 (4), pp. 868-870. Available at https://asmedigitalcollection.asme.org/tribology/article-abstract/125/4/868/462260/Simplification-of-Dynamic-Capacity-and-Fatigue.
- Ibid.
- Harris, T., Rumbarger, J. and Butterfield, C. (2009), “Wind turbine design guideline DG03: Yaw and pitch rolling bearing life technical report,” National Renewable Energy Laboratory. Available at https://www.nrel.gov/docs/fy10osti/42362.pdf.
- “Precessing bearing increases life and load rating,” Design News. Available at https://www.designnews.com/precessing-bearing-increases-life-and-load-rating-0.