Additive manufacturing (AM) has been around for a little over 30 years. It brought agile development into the manufacturing world because companies could now rapidly prototype. There have been substantial scalability issues leading to no significant changes in traditional industrial manufacturing practices. However, this is rapidly changing.
The introduction of new technologies, better simulation, and a greater industry adoption is giving rise to a focus on quality, speed, repeatability, and material properties. This is fueling growth from both internal and external investment into the AM market [6].
According to Wohler’s Report, “The total industry estimate of $7.336 billion excludes internal investments from the likes of Airbus, Adidas, Ford, Toyota, Stryker, and hundreds of other companies, both large and small. A surprising number of the $1-5 billion companies — many of which are unfamiliar to most of us — are investing in AM R&D” [12].
This demonstrates the shift of industry into agile manufacturing, and AM has the ability to dominate rapid prototyping, rapid tooling, mass customization, and onsite manufacturing [10, 11].
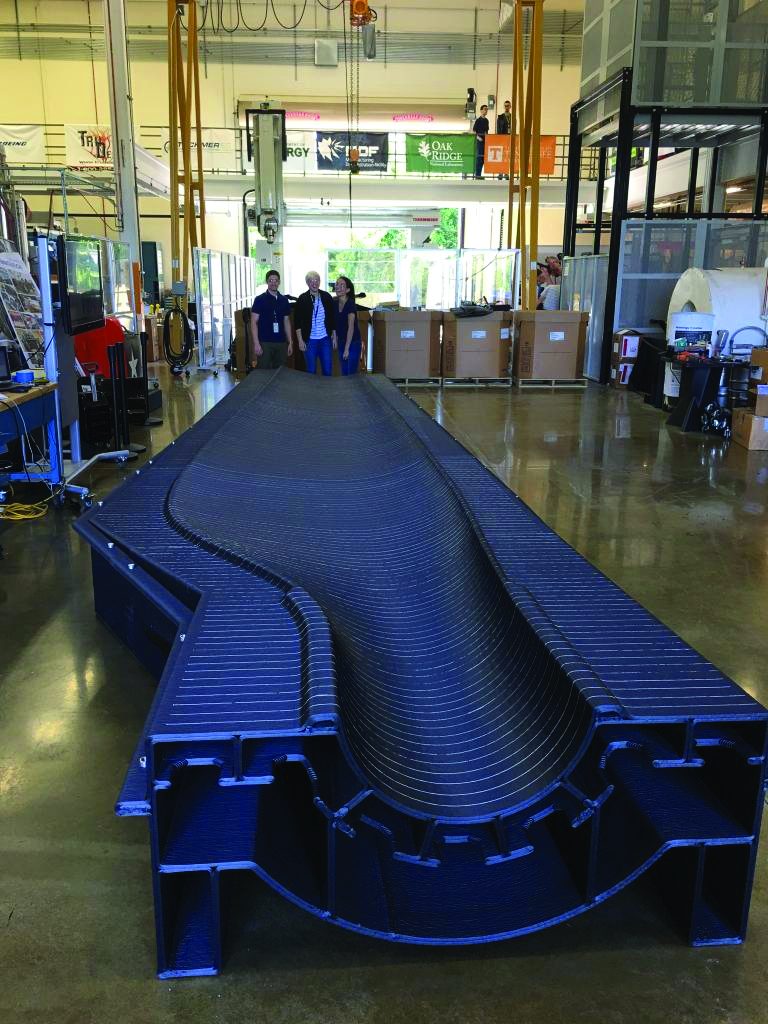
Users and Uses
Each of these uses has notably different and sizable impacts on entire companies and their business models. In a traditional business, AM directly affects product development, manufacturing practices, and inventory with second ripple effects on accounting and supply chain. Startups and established companies alike are recognizing the power of mass customization in manufacturing where each and every product is custom [4]. An example of this in consumer markets is in the eye glassware market, where companies are introducing custom 3D-printed glasses [1].
In the wind-power industry, this could mean optimized wind-turbine blades per tower in a wind farm. In other words, the blades of each turbine can be optimized for the individual location, wind, and turbulence patterns at each and every location in the farm. Additive manufacturing is the technology that makes all this possible at a lower price-point and with shorter lead times [3].
Repair is another region where additive manufacturing can make an impact.
When blades need repair or reach end-of-life, there may be no tooling available to repair or replace the part. AM can be used to make short-run production tools at an affordable price and on-demand.
Rapid prototyping is currently being used to build and test new designs. Printed parts are entering wind tunnels as cheap and easy alternatives to composite molded or machined parts [3]. Similar parts are also being used as molds and fixtures making composite rapid prototyping possible.
In production, rapid tooling makes agile manufacturing feasible. Outfitting a production plant with jigs, fixtures, tools, and molds is now fast and has much less overhead which also means changing practices, products, and designs has a much lower opportunity cost.
The last use category of additive manufacturing is final products. The medical industry has been using AM printed parts for nearly a decade. Two examples are the affordable and highly customizable open-source prosthetics parts made by companies such as the Open Hand Project and in 3D-printed hip replacements [9]. In aerospace, 3D-printed parts can be found across Boeing’s 787 Dreamliner [2]. There are even companies doing on-site production of full-size structures out of concrete such as wind-turbine towers [5].
Case Study
In 2017, TPI Composites concluded a joint research project with Oak Ridge National Labs (ORNL) and Sandia National Labs (SNL). The collaboration demonstrated the feasibility of large-scale AM in reducing production cost, time, and environmental impact of wind-turbine blade molds [3]. Because AM specializes in rapid prototyping, rapid tooling, mass customization, and onsite manufacturing, it met the requirements of the Department of Energy (DOE) [8].
A 2017 DOE report reflected the interest and goals. It stated, “increasing reliability while lowering production costs [are key to] promoting a domestic industry able to meet manufacturing demands while competing globally” [8].
Part of that global competition is the prototyping phase where this study proved the feasibility in prototyping wind-turbine blade molds. As an industry, we are looking to build bigger blades. To do this, we must effectively apply new manufacturing techniques and technologies. Additive manufacturing offers a faster, more efficient, and far more flexible manufacturing option [10], that specializes in the rapid prototyping, rapid tooling, mass customization, and onsite manufacturing; all things critical for future competition and continued innovation.
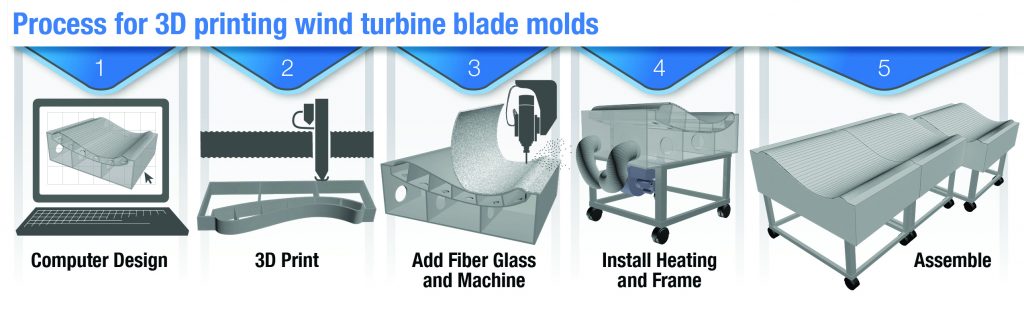
Future Development
Looking forward, there are many challenges for AM to overcome to fully automate the tool-making process and to create 1000-plus cycle molds possible. For the wind industry, surface finish and accuracy are key.
The current producing of a mold with an accurate and fine surface finish requires a complex manufacturing chain very similar to that of current composite molds [3]. As a result, the core offerings of AM — time and cost savings — are nullified by the need for labor, repetitive freight, and multiple companies making margins. Therefore, surface-finish automation techniques and technologies are desperately needed.
Second, more predictable and repeatable prints are a necessity.
Without this, the manufacturing principle of Heijunka or level production occurs.
Heijunka states that no matter what happens, your output remains the same every day; this really means predictability and repeatability [7].
3D-printed parts currently have enough variation in their material microstructure where heat cycling will cause lack of dimensional stability.
Once production-grade parts are achieved, factories and product will be able to rapidly adapt and work in conjunction with digital twins. Wind will power the fourth industrial revolution. Wind-power production should benefit from its advances.
References
- (n.d.). Retrieved from Monoqool: https://monoqool.com/
- Aerospace America. (2018, September). Engineering Notebook: Making 3D-printed parts for Boeing 787s. Retrieved September 2019, from https://aerospaceamerica.aiaa.org/departments/making-3d-printed-parts-for-boeing-787s/
- David. (2018, April 27). Sandia’s first 3D printed wind turbine blade mold wins national Technology Focus Award. Retrieved September 2019, from https://www.3ders.org/articles/20180427-sandias-first-3d-printed-wind-turbine-blade-mold-wins-national-technology-focus-award.html
- Dollarhide, M. (2019, May 23). Mass Customization Definiton. Retrieved from Investopedia: https://www.investopedia.com/terms/m/masscustomization.asp
- Gerdes, J. (2017, November 28). Is 3-D Printing the Solution for Ultra-Tall Wind Turbine Towers? Retrieved from Green Tech Media: https://www.greentechmedia.com/articles/read/is-3d-printing-the-solution-for-ultra-tall-wind-turbine-towers#gs.3wkvqk
McCue, T. (2018, June 4). Wohlers Report 2018: 3D Printer Industry Tops $7 Billion. - Retrieved from Forbes: https://www.forbes.com/sites/tjmccue/2018/06/04/wohlers-report-2018-3d-printer-industry-rises-21-percent-to-over-7-billion/#261e358b2d1a
Mulholland, B. (2018, February 2018). 9 Lean Manufacturing Principles to Kill the Jargon and Get Quality Results. Retrieved September 2019, from https://www.process.st/lean-manufacturing-principles/ - Office of Energy Efficiency & Renewable Energy. (n.d.). Transforming Wind Turbine Blade Mold Manufacturing with 3D Printing. U.S. Department of Energy.
- Olson, P. (2018, March 5). 100,000 Patients Later, The 3D-Printed Hip Is A Decade Old And Going Strong. Retrieved from GE Reports: https://www.ge.com/reports/100000-patients-later-3d-printed-hip-decade-old-going-strong/
- Printing, T. W. (2018, December 21). Office of Energy Efficiency & Renewable Energy. Retrieved September 2019, from https://www.energy.gov/eere/wind/videos/transforming-wind-turbine-blade-mold-manufacturing-3d-printing
- U.S. Food and Drug Administration. (2018, September 27). 3D Printing of Medical Devices. Retrieved September 2019, from https://www.fda.gov/medical-devices/products-and-medical-procedures/3d-printing-medical-devices
- Wohlers. (2018). Wohlers Report 2018. Fort Colins : Wohlers Associates.