Currently, the strength of a large direct drive wind turbine generator (DD/WTG) tower is achieved using various grades of steel that can affect the weight and cost of the structure. The lower the grade of steel, the lower the cost but the higher the weight. A higher grade of steel can reduce the weight of the tower but at a much higher cost. Offshore WTG tower codes call for thicker and stronger grades of steel that can bring the minimum average weight of a steel tower for a 15 MW DD/WTG to well over 1,000 tons. To achieve the goal of lower cost renewable energy, the offshore wind industry needs to evolve toward using lighter, stronger materials for these larger DD/WTG tower structures and adopt more efficient construction methods to reduce costs and ensure environmental sustainability.
New Materials for Marine WTG Towers
Rather than the current practice of using steel rebar and concrete made from ordinary Portland cement (OPC), strength and durability can be increased, and overall weight significantly reduced, using an emerging new class of marine concrete using basalt (generic solidified volcanic rock) rebar and geopolymer cement. Compared to a similar diameter steel rebar, basalt rebar is seven-to-nine times lighter while providing the same strength. Since basalt is stone, there is no cover requirement; it is waterproof, chemically resistant, and fireproof. The tensile strength of basalt rebar is three times stronger than steel rebar, resulting in a smaller diameter rebar of equivalent strength, reducing the weight of the completed product.

Geopolymer cement (GPC) is made up of four inexpensive and widely available components: type-2 fly ash, fresh water, waterglass (sodium-silicate), and lye (sodium hydroxide). Fly ash has as little as 2 percent calcium, producing a saltwater-resistant material that is durable and fireproof. Environmental sustainability is important for clean energy initiatives, and GPC results in an 80 percent reduction in carbon dioxide emissions compared to the production of OPC, while sustainably reusing “waste” materials such as fly ash in its composition. Workability is a major concern in the mix flow of GPC due to its high viscosity. This is easily remedied with the use of a new type of superplasticizers made from rice husks. The structural design of the DD/WTG tower will use basalt rebar for tension requirements and basalt fiber for structural composite reinforcing. The GPC binds to the basalt rebar and fiber on both a chemical and mechanical level to provide the strength characteristics required for this new generation of WTG tower.
Clean, hard, strong aggregate particles will account for 65 to 75 percent of the concrete mix design and will be divided into two distinct categories: fine and coarse. The fine aggregates will consist of granite saw dust, washed and sand sized; and chopped quarter-inch basalt fiber. The coarse aggregates will be crushed granite particles ranging in size from 1/4 to 3/8 inch in diameter. Using both fine and coarse aggregates requires a larger quantity of GPC content that will positively influence the concrete hardening properties, resulting in a stronger final product.
Tower Design, Construction, and Assembly
The geopolymer cement (GPC) and basalt rebar tower for a 15-MW direct drive wind turbine generator (DD/WTG) will be comprised of four sections: three will be constructed of GPC concrete and the fourth one of steel. Due to the consistent viscosity of the GPC mix, slipform production becomes the preferred method for the tower construction. Construction of the three tower zone sections can be done on-site in the back harbor, and because all three sections can be slip-formed at once, the entire process can be completed in one week. The construction of most steel towers strong enough for a 15 MW DD/WTG are primarily being constructed in Spain and Russia, resulting in exorbitant freight costs to the United States.
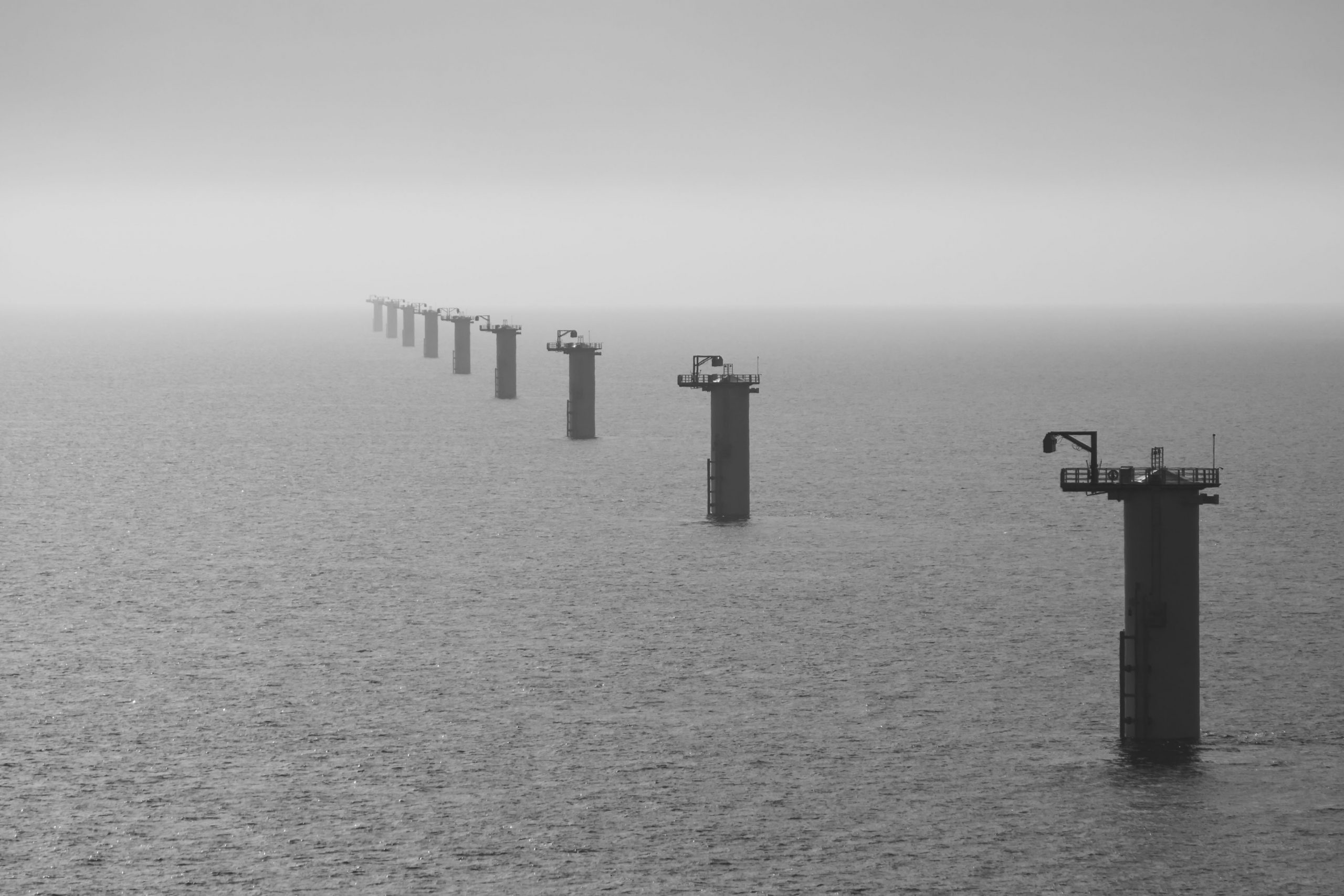
The three concrete tower zone sections are each 121 feet, 4 inches long. Slipform construction will allow for the precision tapering of the outer dimension of the overall tower structure while, at the same time, reducing the wall thickness, resulting in a reduction of weight and subsequent material costs. The use of GPC concrete and basalt rebar and aggregates allows for a stronger and significantly lighter tower design. In addition to the reduction in weight, the high-strength GPC concrete will act as a means of absorbing a variety of vibratory characteristics of the tower, including the natural frequency of the blade pass, the damping ratio, and vibration mode. The three concrete tower zone sections include a base zone weighing 164 tons, a middle zone weighing 107 tons, and an upper zone weighing 63 tons for a total completed tower weight of 334 tons. The result is a tower one-third the weight of a comparably sized steel tower.
Once slip-forming is complete, the tower zone sections are moved from vertical to horizontal to allow for the efficient installation of the internals. These lighter concrete tower zone sections will greatly reduce the craning costs of any handling of the tower zone sections. Assembly at sea can be optimized by eliminating the expensive bolt-up flange plates, which are the key joining components used to connect traditional steel tower sections. The GPC concrete tower zone section flanges are milled for high exactness. The grinding technology necessary to achieve this high level of exactness between the mating surfaces is achieved using computer-numerical-controlled grading. High-strength bull-nose basalt alignment pins are then installed at each end of the concrete tower zone sections. These alignment pins afford a safe and simple process for assembling the tower zone sections: four pins align the sections, and four additional sheer pins provide stability. The precision flanges of the concrete tower zone sections will be held together by the high stress forces of post tension cables (168 tons), plus the weight of the tower aloft of the rotor, hub, and nacelle (784 tons).

Eight integrated slip-formed stanchions are equally spaced on the inside face of each of the tower zone segments. These stanchions serve four important functions:
- They increase the section modulus of the tower structure for added strength.
- They support a three-inch continuous hole tangent to the face of the inside of the tower.
- This hole, after extraction of the slip-formed jack pipe, will serve to house the post tension cables that stress the tower zone sections together.
- The center of each stanchion includes an inch and a half hole for supporting the basalt bull-nosed alignment pins.
- On either side of the stanchions, four inches from the bottom end, are one-inch-deep internal grooves that support the clamps that hold the internals in the tower.
On top of the three concrete tower zone sections is the steel yaw adapter component for the turbine mounting connection. This steel yaw adapter component also serves as the dead-end supports for the post tension cables. These cables, along with the basalt bull-nose alignment pins, serve to align all the tower zone sections together accurately. The installation of the internals and the assembly of the tower takes one week. Including the one week required to slip-form the three tower zone sections, the total build out of the tower is accomplished in just two weeks.
Tower Market, Structural Codes, and Standards
More than 85 percent of the proposed offshore wind farms around the world are out of the range of fixed bottom support structures, creating a market for innovative floating systems, with a new emphasis on those that can support large 15-MW direct drive wind turbine generators.
Until recently, European wind turbine generators’ (WTG) original equipment manufacturers had initially dominated the global wind-energy industry with steel tower structures. Now, there are companies in the U.S. and Europe building tower support structures in engineered wood and precast concrete. The code requirements for fixed bottom WTGs in the U.S. offshore market are well defined and were developed jointly by the American Society of Civil Engineers (ASCE) and the American Wind Energy Association (AWEA) and are collectively known as ASCE/AWEA RP2001. Floating WTGs have their own inherent design complexities, and the International Electrotechnical Commission (IEC) has developed IEC 61400-3 design standards for offshore WTG support structures. The aforementioned codes are minimum standards providing an opportunity for the U.S. offshore wind industry to innovate and develop higher standards using new materials and more efficient production methods.
Cost Savings Associated with GPC Concrete Tower Construction Efficiencies
The design and implementation of a geopolymer cement (CPC) concrete and basalt rebar tower suitable for a 15-MW DD/WTG results in a cascade of cost savings and other benefits throughout the production of a floating offshore system:
Construction takes place at a back-harbor location and does not require the remote construction plant or associated freight costs of a comparably sized steel tower.
The precision tapering of the overall tower results in a reduced wall thickness resulting in reductions in weight and associated material costs.
The GPC concrete and basalt rebar tower weighs one-third the weight of a similar sized 1,000-ton steel tower, resulting in a huge cost saving in the construction of the tower as well as the 650-ton reduction in the supporting floating hull structural capacity.
The cost of craning, transport, and deployment handling of these lighter concrete tower components will be substantially reduced.
The efficiencies of slip-forming all three tower zone sections at the same time, and installation of the internals while the tower zone sections are horizontal, result in substantially reduced labor costs and culminate in a total build out in just two weeks.
The precisely milled concrete tower section joints eliminate the tri-annual maintenance costs normally associated with torqueing the bolt-up flanges of a steel tower.
The lack of steel in the three tower zone sections, along with the durability of the CPC concrete and basalt rebar assure a longer life of the tower (a minimum 100 years).
A preliminary cost opinion by an independent source estimated a savings of more than $1.75 million per tower when accounting for all costs associated with the materials, processes, and labor associated with construction of a 15 MW DD/WTG tower with GPC concrete and basalt rebar compared to a steel tower.