Renewable wind and solar technologies are bringing power to millions across the world with little-to-no adverse environmental impacts. There are a significant number of large new offshore wind farms due to come online over the next few years, and the overall capacity of all wind turbines installed worldwide by the end of 2018 reached 600 GW, according to preliminary statistics published by WWEA2018.
As the installed capacity of renewables increases and is adapted into more energy infrastructures worldwide, it will impose downward pressure on wholesale energy prices. Since wind and solar generation is not baseload or dispatchable, energy-storage solutions are needed to harness the full potential of their output.
One option is a battery energy storage system that stores energy and returns the stored energy as electrons to the power grid. While this approach can help integrate renewable generation and firm intermittent output, it is limited to the power sector and, of course, once a battery is fully charged, its ability to store more is tapped out. Hydrogen as an energy storage medium provides an alternative pathway that, not only helps to integrate renewable power generation, but also enables the decarbonization of the transportation and natural-gas sectors.
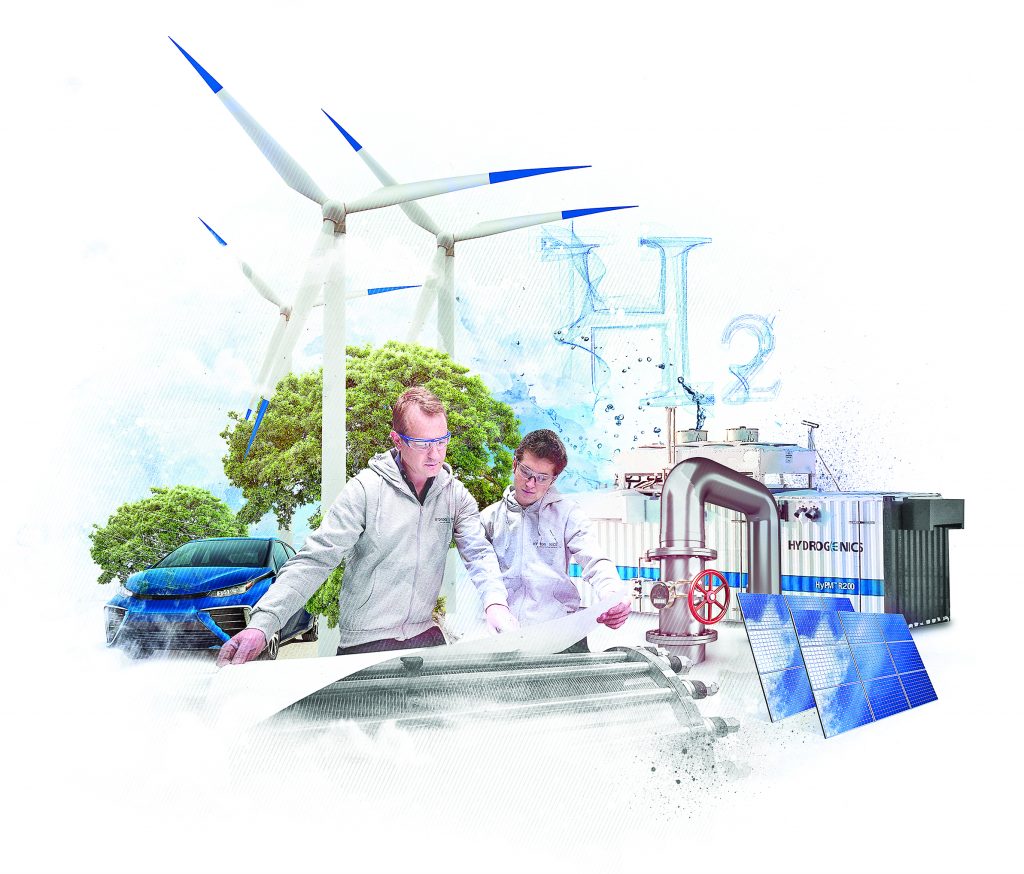
This is accomplished by using an electrolyzer to split water into hydrogen and oxygen, and since an electrolyzer can handle a very dynamic load, it can also be used to provide ancillary power services. There are several ways to commercialize renewable hydrogen generation into the energy system. Hydrogen can be used as fuel for fuel-cell vehicles such as buses, trains, and cars (power to mobility). It can be directly injected into gas grids (power-to-gas), and it can also be used in industrial applications (power-to-industry) in fuel production, or in the production of green chemicals such as methanol and ammonia.
Hydrogen fueling (power to mobility)
Momentum is building for hydrogen fuel cell electric vehicles with clear 2020 and 2030 targets in national and regional policies across several regions of the world including Europe, China, California, and Japan. The largest potential is for bus, truck, and commuter-train fleets to be powered by hydrogen in the short term. In this scenario, excess or dedicated wind power can be used to generate hydrogen with an electrolyzer to be used as fuel for hydrogen fuel cell electric vehicles (FCEV). A FCEV is an electric vehicle in which a fuel cell is used to keep a small battery at an optimal rate of charge. The drivetrain of a FCEV is the same as a battery electric vehicle. A FCEV provides zero emission transport with the same performance in terms of range, payload and refueling time as a conventional vehicle. Since fuel cells are able to deploy energy in very dense packages, they are also quite suited to moving heavy duty vehicles.
A case in point: Alstom has developed a fuel cell electric version of its Coradia LINT passenger train, and it is now in public service in Germany. The Coradia LINT provides the same performance and operational capabilities as its conventional diesel version. Several bus manufacturers and integrators in China, Europe, and North America have rolled out fuel-cell electric buses. California has supported the build-out of a critical-mass network of hydrogen-fueling stations in southern California for fuel-cell electric automobiles, and there are now more than 6,500 FCEVs on the road. The potential future demand for renewable hydrogen fuel as the FCEV market grows is enormous.
Vehicle refueling is among the fastest-growing uses for hydrogen with a growing preference for green hydrogen due to policy mandates. There are more than 300 refueling stations in operation today, with at least 100 stations expected to be added annually through 2025.

Renewable natural gas (power to gas)
By adding hydrogen to the natural gas grid, energy storage is expanding to the TWh range. There are two techniques for this: direct injection into the natural gas grid and conversion of hydrogen into synthetic methane (methanation). In direct injection, pure hydrogen enters the gas grid typically at up to 1- to 10-percent concentration (depending on the applicable regulation).
Even with a 2-percent limitation, the potential storage capacity of natural-gas grids is immense. Methanation overcomes direct injection’s limitations, producing methane that is fully compatible with natural gas and that can be added directly to the gas grid without limitations.
Power-to-gas energy storage may be one of the more cost-effective ways to reach the targets for climate protection in the long term by using existing infrastructure for large scale conversion of renewable energy. Moving and storing renewable hydrogen and methane in gas pipelines reduces the cost of switching to renewable energy as well as the need for new infrastructure. Many gas networks have storage caverns and/or infrastructure whose capacity could be used to store the hydrogen. This hydrogen can be later used to run gas-fired electric generation power plants during periods of low wind and solar output using the existing gas infrastructure.
Power to industry
Hydrogen is also used by refineries, power plants, and many industrial processes including steel and metal processing, glass, oil and fat hydrogenation, and electronics manufacturing. In this scenario, excess wind energy can be used to generate hydrogen that can be commoditized for use in the production of products or the refinement of fuel. Today, more than 43 percent of global hydrogen production is used in refineries to produce and remove sulphur from fossil fuels. But the hydrogen is usually produced from the reforming of natural gas which generates around 10 metric tons of CO2 for each metric ton of H2.
The concept replaces this hydrogen with hydrogen that is generated from renewables like wind, considerably reducing the carbon footprint of the conventional refineries. In this situation, wind energy can be used to replace traditional fossil fuel as well as reduce the carbon output of existing fossil fuel-based energy.
There is growing interest in the production of green chemicals such as methanol and ammonia using renewable hydrogen instead of natural gas as a feedstock. For methanol production, renewable hydrogen is combined with carbon dioxide captured from industrial processes or the atmosphere. For ammonia production, renewable hydrogen is combined with nitrogen.

Ancillary services
An electrolyzer can operate as a fast-responding, dynamic load whose power consumption can be changed rapidly over its operating range in response to a signal from an independent system operator (ISO). This enables it to provide a frequency regulation service that helps the ISO correct for short-term variations in the frequency of the power system resulting from changes in the balance of electricity supply and demand. The regulation signal is typically adjusted every two seconds. The load of an electrolyzer also can be used to modulate the output of an off-grid wind farm by absorbing excess power as required.
Other ancillary services including frequency response and operating reserve can be provided by an electrolysis system. Operating (non-spinning) reserve is stand-by power or demand reduction that can be called on with short notice to deal with an unexpected curtailment of a generator. An electrolyzer can provide this service by operating at full capacity producing hydrogen and within 10 minutes’ notification, it drops its load to its minimum operating point for one hour as a dispatchable load.
The core function of renewable hydrogen electrolysis is producing hydrogen for a specific application, either hydrogen fueling, renewable natural gas, green chemicals, and industrial applications. Ancillary services are an incremental service and revenue stream and is tailored to the hydrogen demand for the application. It is possible to allocate a portion of the operating capacity of the electrolyzer plant for ancillary services.

More than 35 renewable hydrogen demonstration projects have been built in Europe across all of the applications — hydrogen fueling, renewable natural gas (direct injection and methanation) and industrial hydrogen supply) — and a few projects have demonstrated ancillary services as well. The first multi-MW facility in North America, a joint venture between Hydrogenics and Enbridge Gas Distribution, is under contract with the ISO in Ontario to provide regulation services.
Electrolyzers
The heart of renewable hydrogen electrolysis is the electrolyzer. Electrolyzers are connected to the grid or off-grid source of power and typically the municipal water supply. Other key components are rectified to provide DC power input and a water treatment facility to produce deionized water. Hydrogen and oxygen are liberated by splitting water molecules. Electrolysis for industrial hydrogen applications has been used for decades and deployed onsite to meet the hydrogen demand, typically on a scale of 5 to 30 kilograms of hydrogen per hour. The scale and potential market size for renewable hydrogen is significantly larger. For example, a 10-MW electrolyzer plant would produce enough hydrogen to fuel a single fleet of 130 city buses. Multiple electrolyzers can be combined and scaled in a centralized hydrogen fuel production facility in excess of 100 MW, capable of supplying green methanol or ammonia plants that often require on the order of 100 MW to 300 MW of electrolysis.
Advancements in this technology have been most dramatic for proton-exchange membrane (PEM) electrolyzers. The price of PEM electrolyzers has dropped significantly during the past decade by roughly 40 percent, according to a study published in February in Nature Energy. A PEM electrolyzer is up to five times more compact than an alkaline electrolyzer of the same capacity and provides the ability to vary its power consumption within seconds to match the output of a wind farm.
Leveraging depreciated wind-farm assets
In jurisdictions such as Germany and California, which were early adopters of renewable generation, a growing number of wind-farm operators have reached the stage where their contracted feed-in-tariff has expired. However, there is often still plenty of life left in these assets. The option of selling power into the spot market is available, but in some jurisdictions, the market power price is often low (and at times negative) at times of peak wind generation.
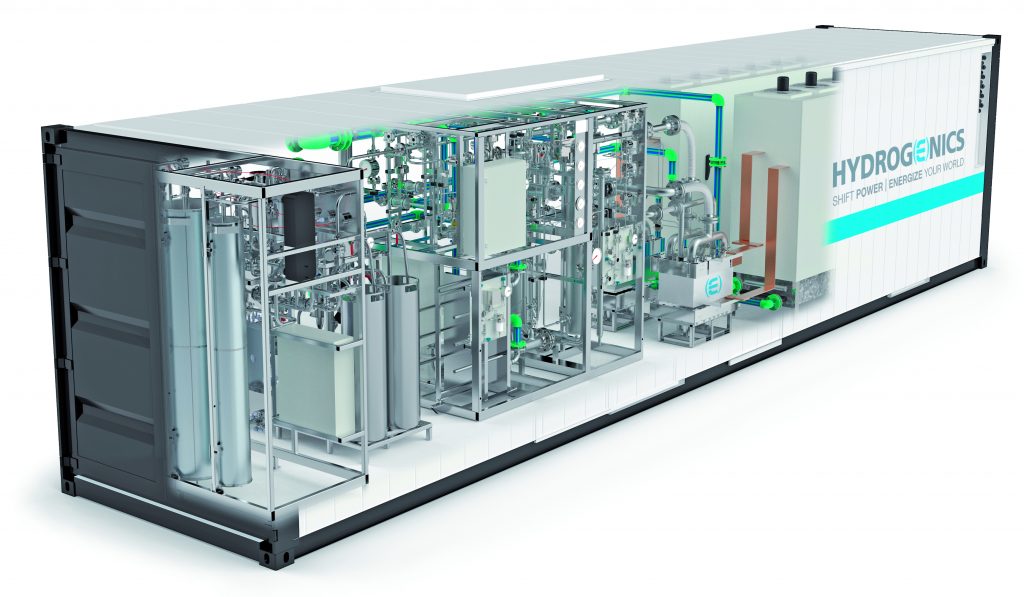
But the ability to produce electrolytic renewable hydrogen with these wind assets is becoming more and more of a viable option as well. California requires 33 percent of the hydrogen used for fueling FCEVs be from renewable sources. Europe is in the process of adopting renewable targets for transportation fuels and developing standards to measure and track the renewable content of hydrogen under the CertifHY program. There is significant potential in the Middle East, North Africa, and the North Sea as they generate renewable energy to serve Europe and other regions. The Western U.S. and Canada have the potential to become a hydrogen trade hotspot, with cheap hydropower, an aggressive renewable portfolio, and decarbonization goals, and an increase in recent hydrogen investment activity. There are numerous market growth indicators at play, and the market for renewable hydrogen is expected to continue its growth.
Hydrogen is well positioned to play an important role in the transition to decarbonized energy systems with the integration of FCEVs, improved technology efficiency, and decreased technology costs, and the ability to commoditize, package, transport, and store energy with hydrogen for a variety of purposes and applications. All these factors are leading to growing demand for renewable hydrogen worldwide. Renewable hydrogen produced by electrolysis from wind and solar will be used to fuel buses, trucks, and trains; produce renewable natural gas; and produce green chemicals and feedstocks. It provides a pathway to leverage large scale wind farms to not only decarbonize traditional power markets but also the transport, natural gas, and chemical sectors. And it’s already under way. Many major companies around the world are strategically transitioning to hydrogen generated from renewable sources to help reduce their carbon footprint. The value of renewable wind energy can now be captured, stored, and monetized as never before.